An article by Werner Wöhrmann, Olaf Homeier, Frederik Gerdes, Thomas Grunge,
Dr. Gerold Holtkamp and Dr. Achim Tegeler in December 2024
Anyone who has ever been able to enjoy the view through a telescope at an observatory or, for example, during our magic hours, has certainly been able to admire a globular cluster.
These objects, which are barely perceptible to the naked eye, turn out to be true jewel boxes in the night sky when magnified accordingly. Hundreds of thousands or even millions of stars standing together in a spherical structure in one spot – often up to over 30,000 light-years away from us. But what exactly are globular clusters and are they all the same?
In this article, we want to take a closer look at these mysterious objects and show the current state of science on globular clusters.
So what are globular clusters?
Globular clusters (GCs) are huge collections of stars with up to several million stars that are gravitationally bound to each other. Typical representatives of these objects are M13 (about 300.000 stars], M15 (about 500.000 stars] or Omega centauri with more than 10.000.000 stars.
GC show an extremely dense distribution of stars in the center of the cluster: The stellar density in the center of a GC is up to a million times higher than in the vicinity of our Sun.
The vast majority of globular clusters that we can see from Earth lie in the so-called halo [1] of the Milky Way. This halo is a huge, spherical area of interstellar matter and stellar streams, in which the rather flattened galaxy is embedded. The halo of the Milky Way has a diameter of 1 million light-years – the even larger Andromeda galaxy is surrounded by a correspondingly larger halo, so that the two galaxies which are 2.5 million light-years apart, practically touch or even overlap with their halos [2].
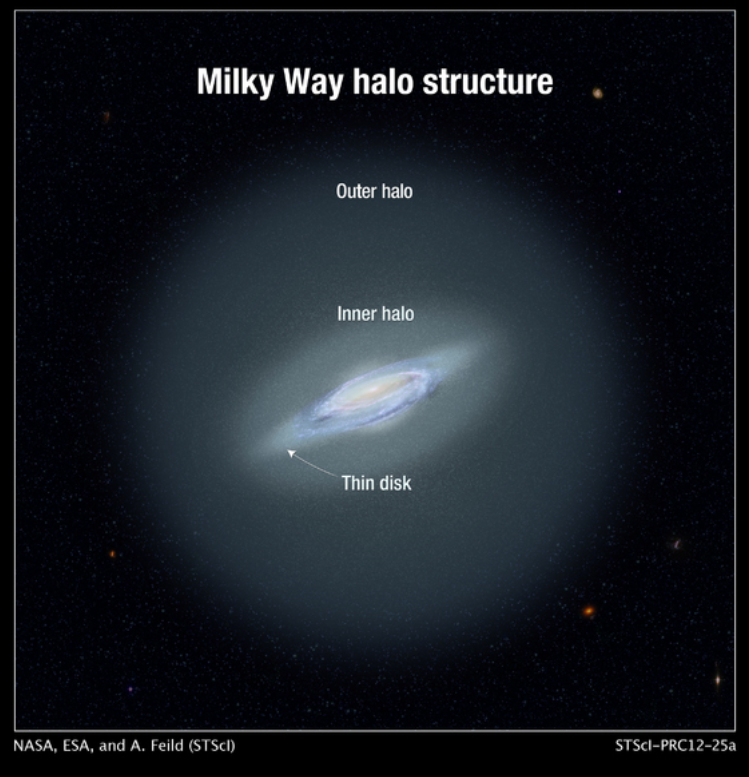
As early as 1979, Cecilia Payne Gaposchkin [3] mapped the globular clusters in the Milky Way and compared the positions with those of open star clusters [4] and found that open star clusters are positioned in the galactic plane, while globular clusters are mainly found in the halo outside the galactic plane.
Globular clusters are also known in other galaxies and can also be observed and photographed by amateur astronomers.
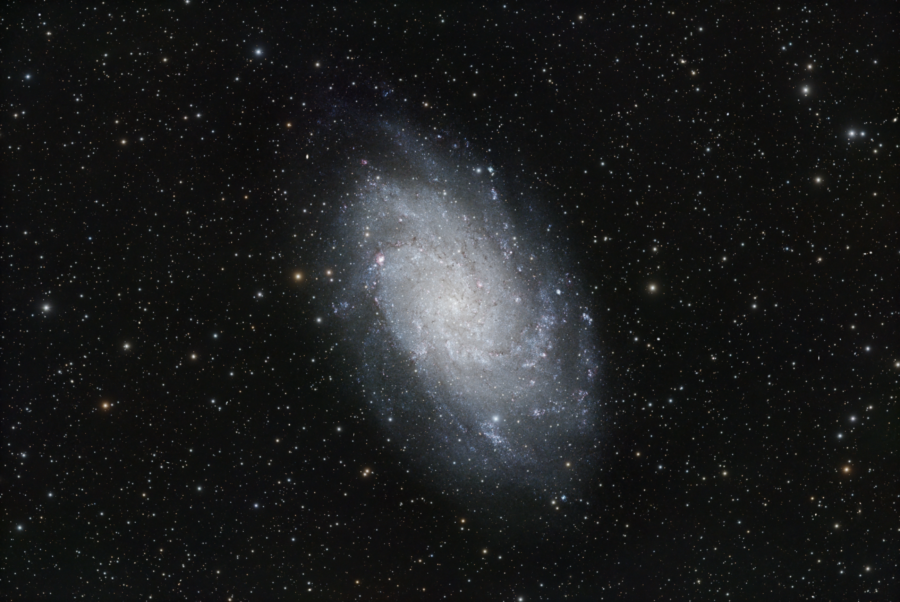
Known GCs in M33 - Info on mouseover. Photo: Olaf Homeier
The age of globular clusters
Globular clusters are very old structures that are between 10 and 13 billion years old. Along with galaxies, they are thus the oldest visible structures in the universe. The age of the clusters can be deduced, among other things, by the so-called turn-off point in the Hertzsprung-Russel diagram. The Hertzsprung-Russel diagram [5] shows the usual distribution of all visible stars plotted by spectral class and brightness.
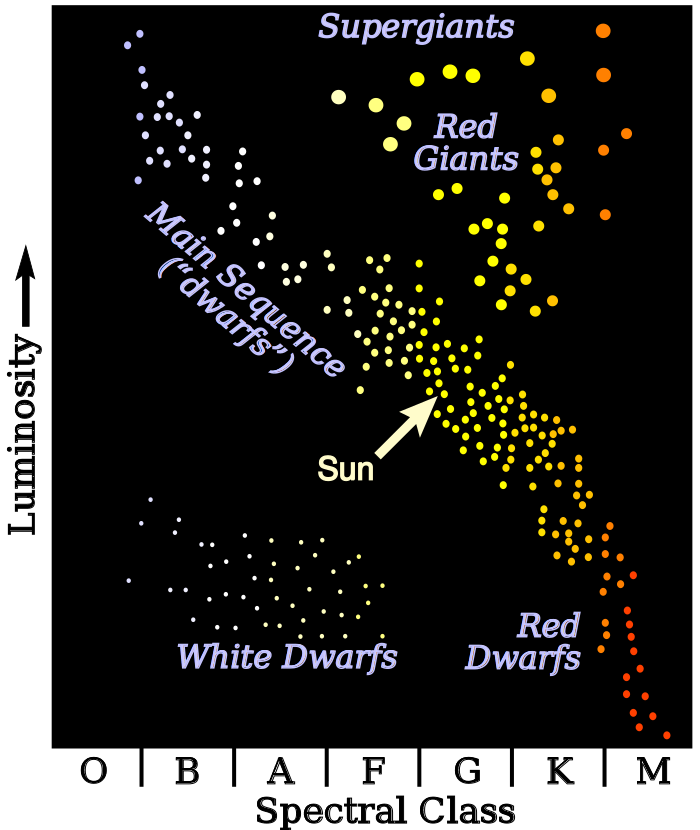
Source: User:Rursus , CC BY-SA 3.0 via Wikimedia Commons
About 90% of the stars lie in the so-called main sequence, which stretches from the upper left (very hot and very bright stars of spectral class O) to the lower right (cooler and less bright stars of spectral class M). This main sequence represents the stars that derive their energy from the fusion of hydrogen into helium. However, the very large, hot stars consume their supply of hydrogen much faster than the cooler representatives of the main sequence, and so the position of a star on the main sequence can also be correlated with a maximum "lifetime" of the star – bright and hot stars exist for only a few million years, while cool M stars can theoretically feed on their hydrogen supply for over 100 billion years.
If you enter the stars of a globular cluster in such a (color-brightness) diagram, you will notice that stars are not present from a certain point in the main sequence. From this turn-off point, the stars have migrated to the right towards the red giant stars. From the position of this turn-off point, the age of the migrated stars can now be calculated. The spectra of these stars also show very low metallicity, suggesting Population II stars, a generation of stars that dates back to the very early phase of the Universe, when hardly any larger elements than hydrogen and helium existed.
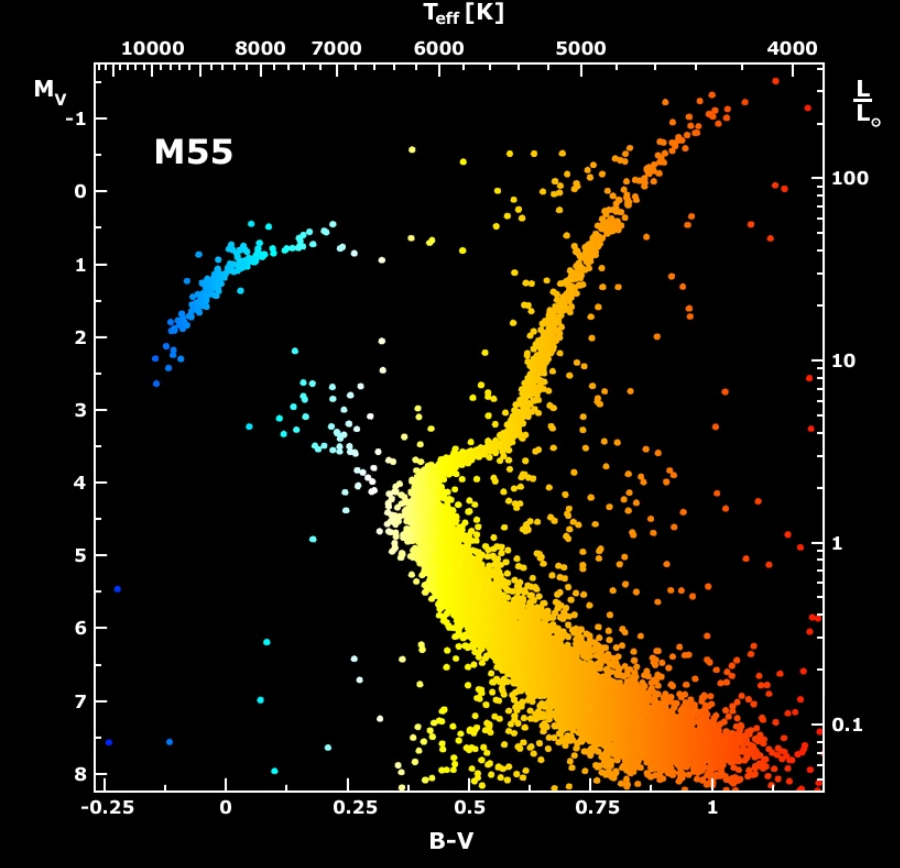
Quelle: https://apod.nasa.gov/apod/ap010223.html B.J. Mochejska, J. Kaluzny (CAMK), 1m Swope Telescope
The distance to Globular Clusters
Distance measurements on astronomical objects are always quite time-consuming, because only the light of the object is available for analysis. The parallax method [6], which allows distances of up to 1 kPc or, in extremes, 5 kPc (about 16,000 light years), is not sufficient for determining the distances of globular clusters. However, similar to the Cepheids characterized by Henrietta Swan Leavitt [7] [8], one can also find periodically variable stars in globular clusters (see also here) finden – die RR Lyrae stars [9]. These variable stars also allow the absolute luminosity to be determined via the period-luminosity relationship [10] and thus a distance measurement. In addition, the period durations of the RR-Lyrae stars characterize the GCs even more closely.
The Oosterhoff classification
The Dutch astronomer Pieter Theodorus Oosterhoff [11] discovered that globular clusters can be divided into two groups, namely those containing RR-Lyrae stars with a shorter period of 0.56 days (Oosterhoff type I) and those containing RR-Lyrae stars with a longer period of 0.66 days (Oosterhoff type II) – the Oosterhoff dichotomy [12]. Type I GCs are described as "metal-rich" and are more likely to be found in the bulge (around the center) of the Milky Way, while Type II GCs are described as "metal-poor" and are located in the halo of the Milky Way. Apparently, the places of origin of the GCs of different Oosterhoff types were originally located in different places, because they have different chemical compositions. However, the Oosterhoff typing is questioned after recent spectral analyses, as intermediate types are also found (Oosterhoff gap) [13], which are not covered by the classical model and which suggest further aspects for the classification of GCs [14].
The Shapley-Sawyer classification
In 1927, Harlow Shapley and Helen Sawyer Hogg made an optical distinction between the globular clusters observed up to that point. In doing so, they divided the GCs into 12 different concentration classes according to the degree of concentration distribution of the stars within the GC that can be seen in the photos at the time [15]. This classification is still of particular interest to amateur astronomers today, because it reflects the optical impression that a GC in the telescope can be expected. Interestingly, the Stellarium software, which is used by many, also includes the Shapley-Sawyer concentration class [16] of GCs in the detailed description.
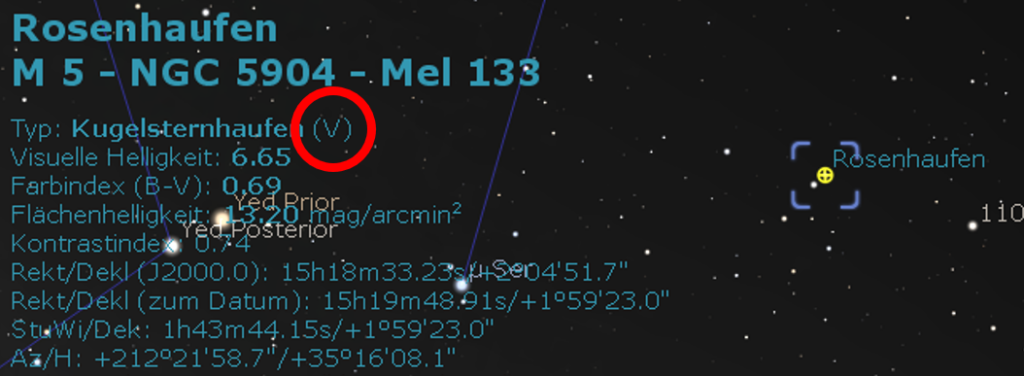
Different origins of globular clusters?
The different appearances in age and composition and different locations of globular clusters in relation to the galactic disk suggest that the clusters could be of different origins. It is therefore being discussed whether globular clusters could be remnants of dwarf galaxies that have collided with our galaxy (merger) and have retained their core in the form of GCs. Such collisions have apparently occurred several times (Kraken (v. 11 billion years), Gaia-Enceladus (9 billion years), Helmi, Sequoia, Sagittarius (7 billion years old – not yet completed)), and the stellar streams originating from these collisions are currently being investigated and simulated with simulations (E-MOSAICS simulations) on mainframe computers [17]. At least for M54, it has been proven that this GC represents the remnant of the merger between the Milky Way and the Sagittarius dwarf galaxy [18]. The GC M56 [19] for example, runs retrograde to the direction of rotation of the Milky Way and therefore hardly allows any other conclusion than that this GC (in the form of a dwarf galaxy?) has penetrated the galaxy from the outside. The easily detectable stellar streams in the halo of the galaxy also suggest this merger scenario [20].
Inside a globular cluster
Due to the great age of KSH, it can be assumed that a large proportion of the stars it contains have left the main sequence and are on their way as red giants in the last part of their existence. Due to the stellar winds of so many stars, there should also be hardly any interstellar medium from which new stars could form. Nevertheless, blue, young stars of spectral type A can also be found in GCs. These Blue Stragglers [21] did not form from molecular clouds, but emerged from stellar collisions of at least two stars of approximately equal mass. Such collisions apparently occur from time to time at such a high stellar density as in the density of a GC. From such collisions and close encounters between the stars in the GC, yellow and red stragglers are also described which, depending on the mass distribution of the stars involved, arise from the transfer of matter from one star to the other.
Interestingly, although the highest density of blue stragglers is in the center of the GC, there is a further concentration of stragglers in the peripheral areas of the GC. Apparently, the blue giants migrate further and further towards the center of the GC over time by releasing energy to smaller stars. Black holes may also be involved in this movement.
Black holes in globular clusters
As early as the 1990s, there was intensive discussion about whether black holes form in globular clusters and can persist there for a long time [22]. In 2007, the first black hole in a globular cluster outside our Milky Way galaxy was discovered in the galaxy NGC 4472 (M49, 4952 million light-years away) [23] by measuring X-rays, the characteristics of which pointed to an accreting black hole [24]. In the following years, more black holes were identified and finally in 2019 several in the globular cluster NGC 3201 [25].
It is now generally assumed that, roughly estimated, the formation of 100 to 1000 black holes in GCs was very common and that they play a special role in the long life of globular clusters. The black holes are formed from the most massive stars, which reach their "end of life" within a few 10 million years and can become black holes. Due to their enormous mass compared to other stars, they migrate to the center of the globular cluster and form a kind of subsystem there.
Later, neutron stars form from the slightly less massive stars, and much later the Sun-like stars also become white dwarfs. Dr. Kyle Kremer presented this development very vividly in a lecture in 2023 [26]:
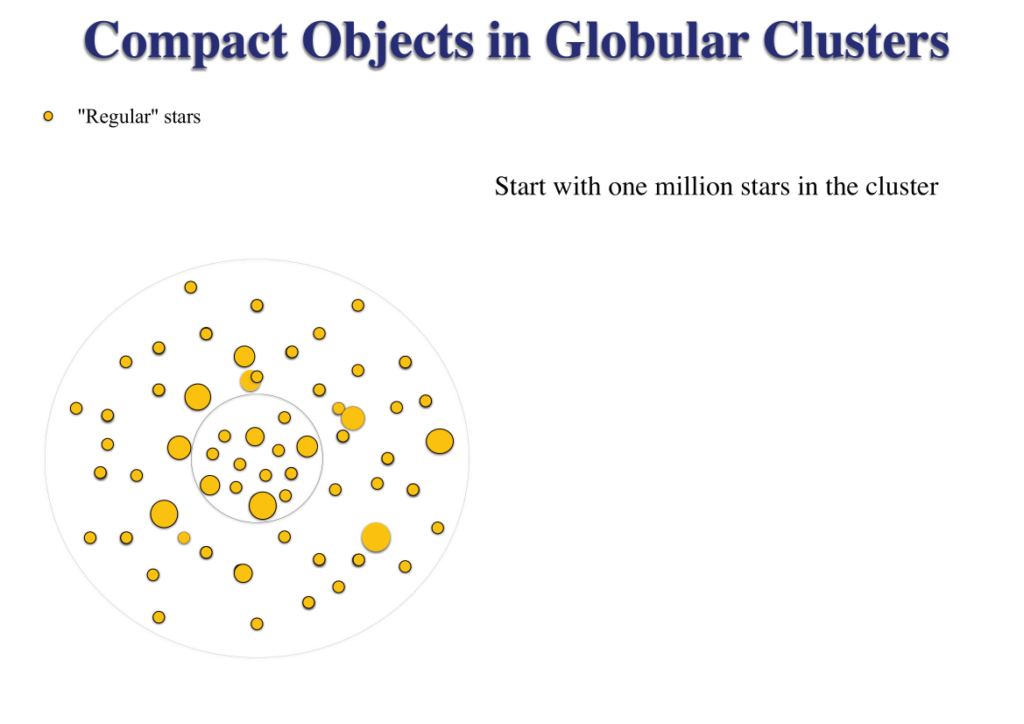

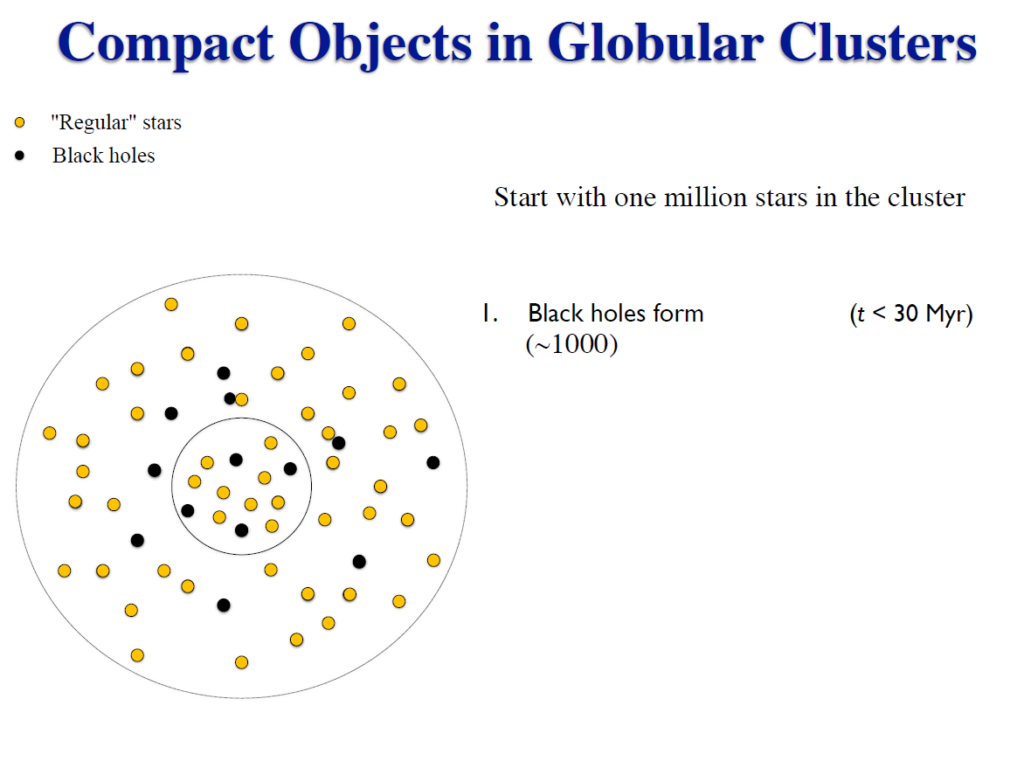

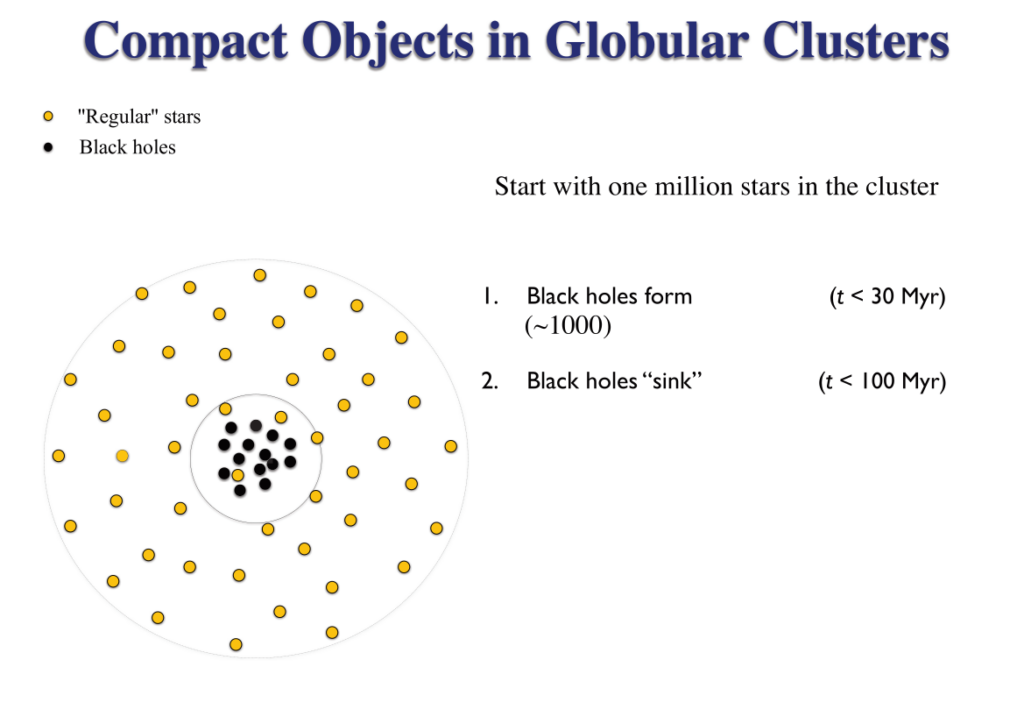

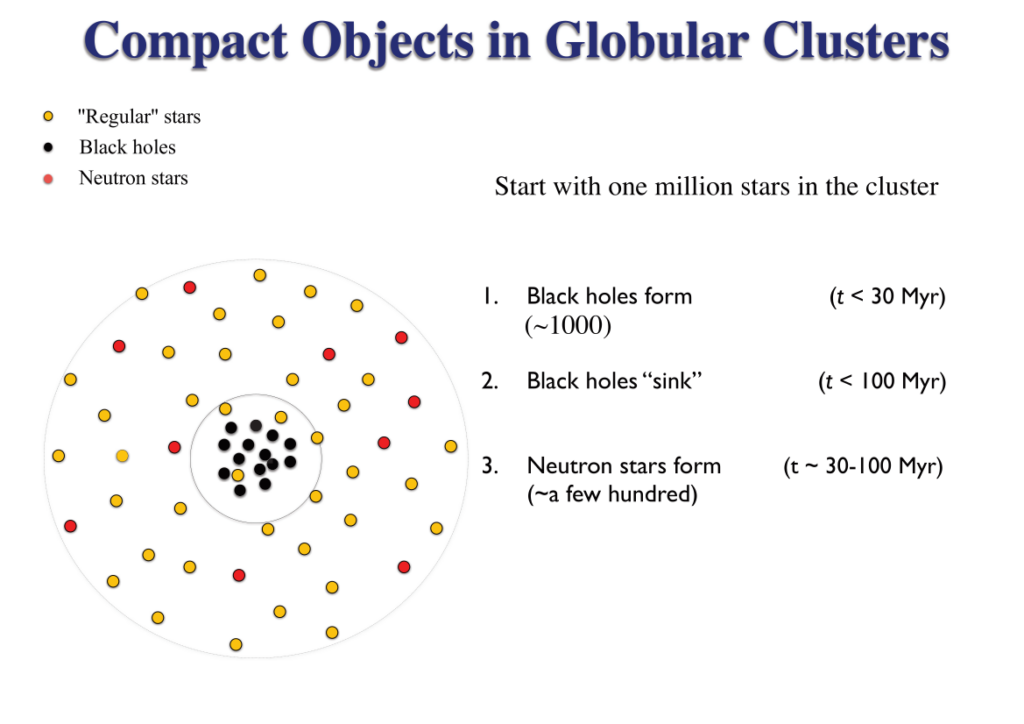

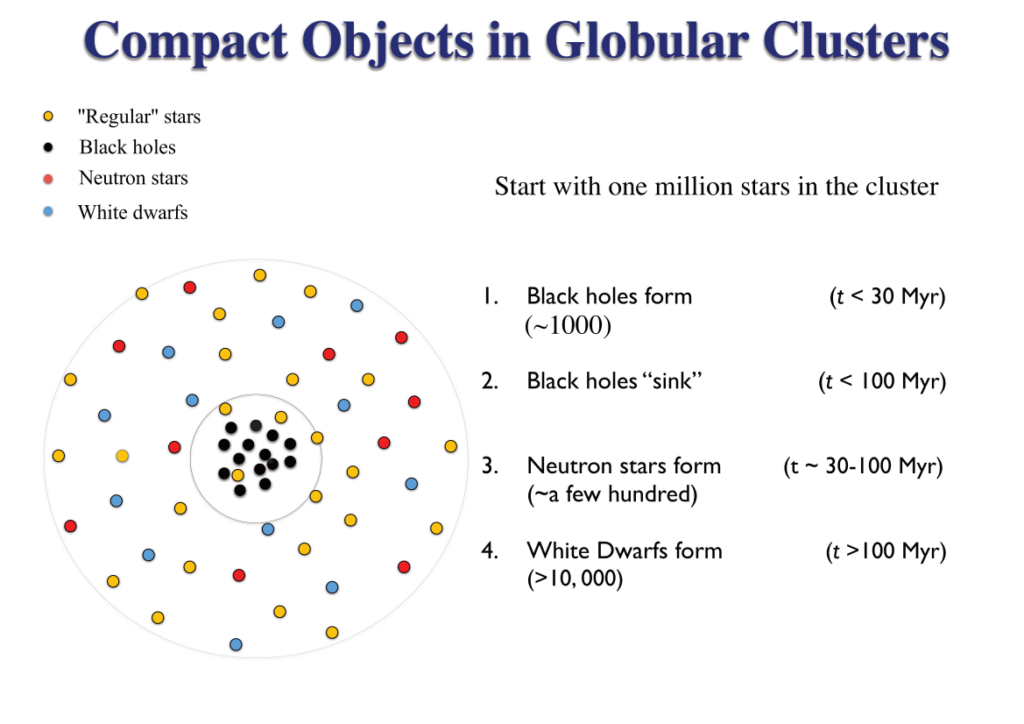
All illustrations courtesy of Dr. Kyle Kremer, UC San Diego
Stars that come close to one of these black holes in the center receive additional energy from this much heavier body through the so-called swing-by effect. As a result, the black hole subsystem "heats" the central region of the globular cluster, so that many stars are there. It can be observed in these globular clusters that the surface brightness coming from the outside no longer increases from a certain distance from the center (about 1 to 2 parsecs). This property is shown by about 80% of all observed globular clusters. In the remaining 20%, the surface brightness increases steadily up to the center – so there is no defined core. They are therefore referred to as Core-collapsed globular clusters.
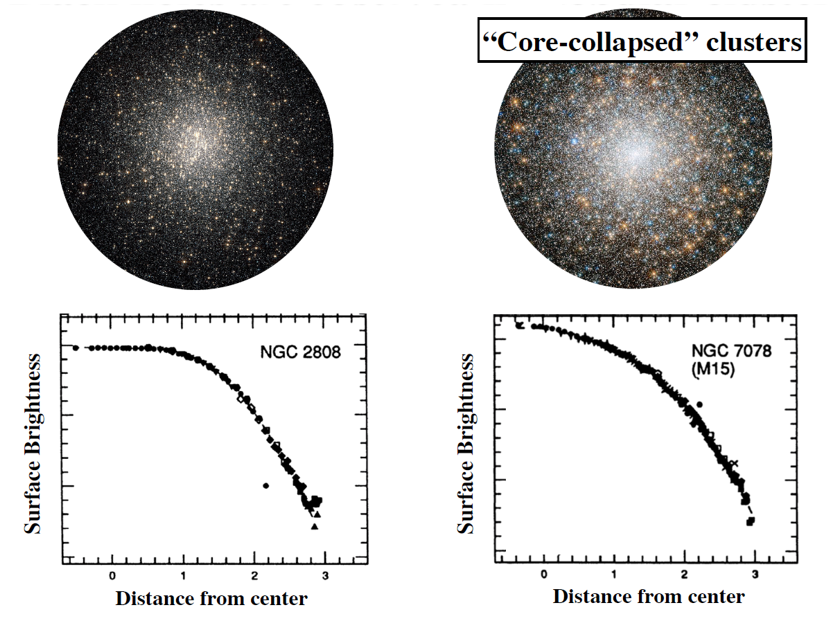
An attempt is made to explain this by the fact that in such globular clusters there are no or hardly any black holes left in the core that can "heat it up". Why the black holes were lost here is the subject of the current discussion. Swing-by events are also suspected here in which the black holes catapult each other out of the GC [27] [28].
Conclusion
With all this information about globular clusters in mind these giants so far away, seem even more enigmatic and fascinating than they already do when viewed through a telescope. Here are some beautiful specimens:
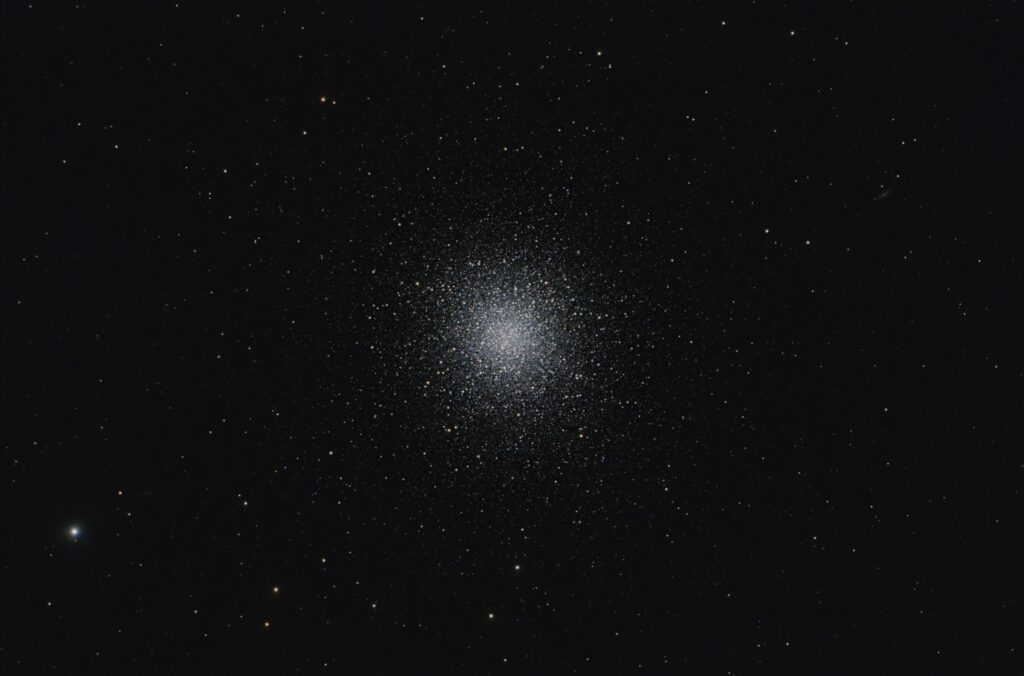
M13 Photo: Olaf Homeier
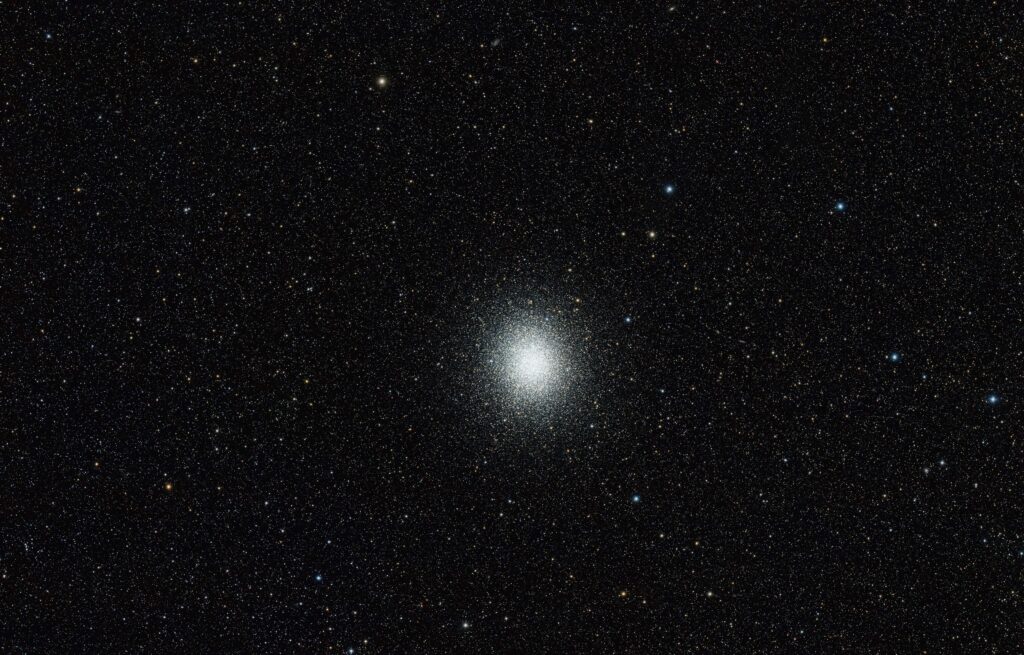
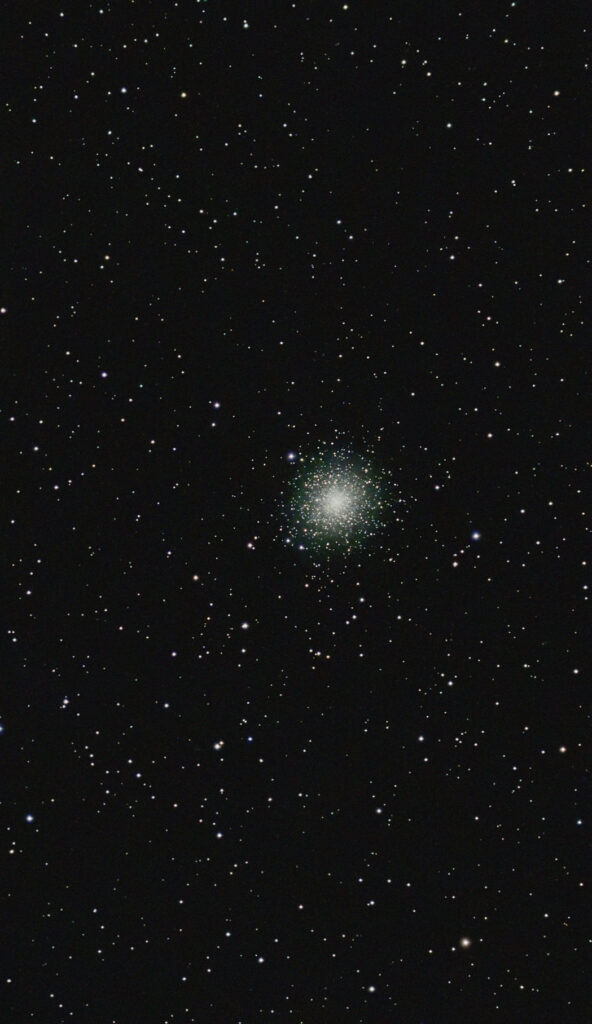
M2 Photo: Olaf Homeier
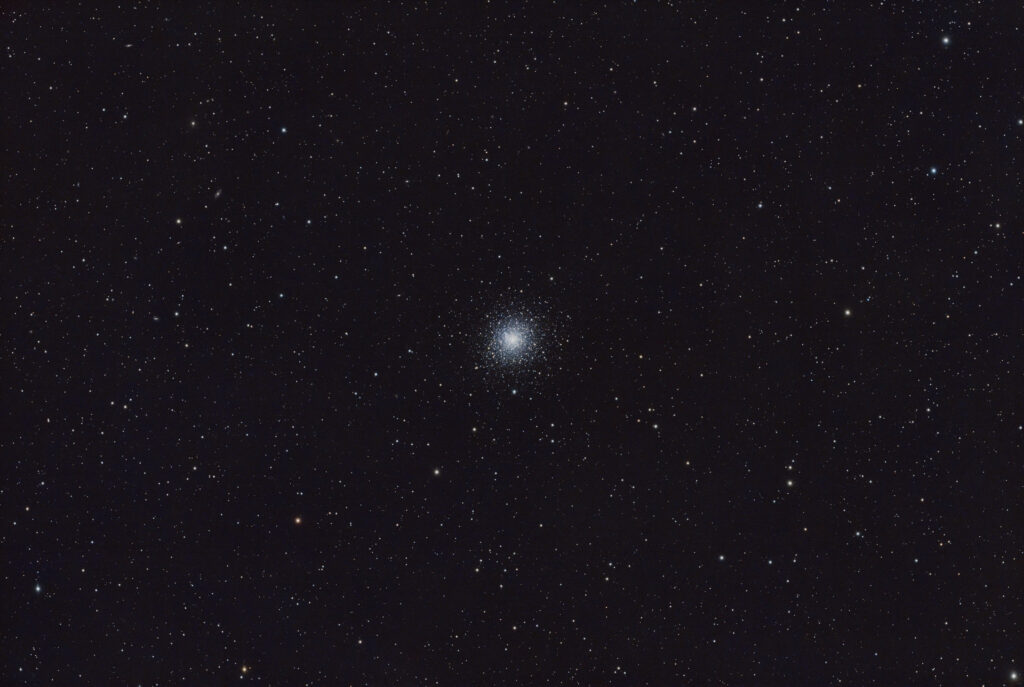
M92 Photo: Olaf Homeier
For all those who prefer to look at the topic of globular clusters audiovisually, we recommend these posts on Youtube:
In English language:
The Dynamic Lives of Stars and Black Holes in Globular Clusters
2023-04-10 Dr. Kyle Kremer
2024-10-06 Dr. Rod Pommier
In German language:
Kugelsternhaufen: Vielfältiger als gedacht!
2022-02-17 Dr. Andreas Koch-Hansen, Haus der Astronomie
Sources:
[1] Galactic Halo https://en.wikipedia.org/wiki/Galactic_halo
[2] Nicolas Lehner et al 2020 ApJ 900 9 https://iopscience.iop.org/article/10.3847/1538-4357/aba49c/pdf
[3] Cecilia Payne-Gaposchkin: https://en.wikipedia.org/wiki/Cecilia_Payne-Gaposchkin
[4] Cecilia Payne-Gaposchkin „Stars and clusters“ in: The Harvard Book of Astronomy, Cecilia Helena Payne-Gaposchkin 1979 Ch.12, S. 159
[5] Hertzsprung-Russel Diagram https://en.wikipedia.org/wiki/HertzsprungRussell_diagram
[6] Parallax https://en.wikipedia.org/wiki/Parallax
[7] Henrietta Swan Leavitt https://en.wikipedia.org/wiki/Henrietta_Swan_Leavitt
[8] Cepheid variables https://en.wikipedia.org/wiki/Cepheid_variable
[9] RR-Lyrae stars https://en.wikipedia.org/wiki/RR_Lyrae_variable
[10] Period-luminosity relation https://en.wikipedia.org/wiki/Period-luminosity_relation
[11] Pieter Oosterhoff https://nl.wikipedia.org/wiki/Pieter_Oosterhoff
[12] Oosterhoff Dichotomie; Oosterhoff, P. T. 1939, The Observatory, 62, 104
[13] Prudil et al. (2024), MNRAS 534, 3654–3664 (2024)
[14] Mauricio Cruz et al. (2024, A&A, 684, A173 (2024)
[15] Shapley, Harlow; Sawyer, Helen B. A Classification of Globular Clusters, Harvard College Observatory Bulletin, August 1927
[16] Shapley-Sawyer concentration classes https://en.wikipedia.org/wiki/ShapleySawyer_Concentration_Class
[17] Kruissen et al. 2020, Monthly Notices of the Royal Astronomical Society, Volume 498, Issue 2, October 2020, Pages 2472–2491
[18] Dissertation Mayte Alfaro Cuello, 2019; The Nucleus of the Sagittarius Dwarf Spheroidal Galaxy: M54
[19] Messier 56 https://en.wikipedia.org/wiki/Messier_56
[20] Rod Pommier, 08.2024 Survivors from the Milky Way’s birth
[21] Blue stragglers https://en.wikipedia.org/wiki/Blue_straggler
[22] R Kulkarni et al. 1993; Stellar black holes in globular clusters, Nature volume 364, pages 421–423 (1993), https://www.nature.com/articles/364421a0 )
[23] Messier 49 https://en.wikipedia.org/wiki/Messier_49
[24] Thomas J. Maccarone et al. 2007; A black hole in a globular cluster, Nature volume 445, pages 183–185 (2007), https://www.nature.com/articles/nature05434 )
[25] Benjamin Giesers et al. 2019; A stellar census in globular clusters with MUSE: Binaries in NGC 3201, Astronomy & Astrophysics 632, A3 (2019), https://www.aanda.org/articles/aa/pdf/2019/12/aa36203-19.pdf
[26] Lecture: The Dynamic Lives of Stars and Black Holes in Globular Clusters, Dr. Kyle Kremer April 10, 2023 https://www.youtube.com/live/BeXS9BlHUP8
[27] Kyle Kremer et al. 2020; Modeling Dense Star Clusters in the Milky Way and Beyond with the CMC Cluster Catalog, The Astrophysical Journal Supplement Series, 247:48 (44pp), 2020 April, https://iopscience.iop.org/article/10.3847/1538-4365/ab7919/pdf
[28] Kyle Kremer et al. 2021; White Dwarf Subsystems in Core-Collapsed Globular Clusters, The Astrophysical Journal, 917:28 (19pp), 2021 August 10, https://iopscience.iop.org/article/10.3847/1538-4357/ac06d4/pdf