A brief overview
Dr. Achim Tegeler, September 2024
In connection with our kosmos-os Excursion to the Effelsberg radio telescope on 21.09.2024 it seemed sensible to take a closer look at the topic of radio astronomy in order to better understand what radio telescopes and thus also in Effelsberg are actually being worked on. Here is a brief overview of radio astronomy.
In addition to classical astronomy (visual telescopes and optics), radio astronomy is another way to perceive the electromagnetic radiation of objects on Earth. However, the Earth's atmosphere only allows certain ranges of wavelengths to pass through to the Earth's surface – and only in these can observations be made from the ground.
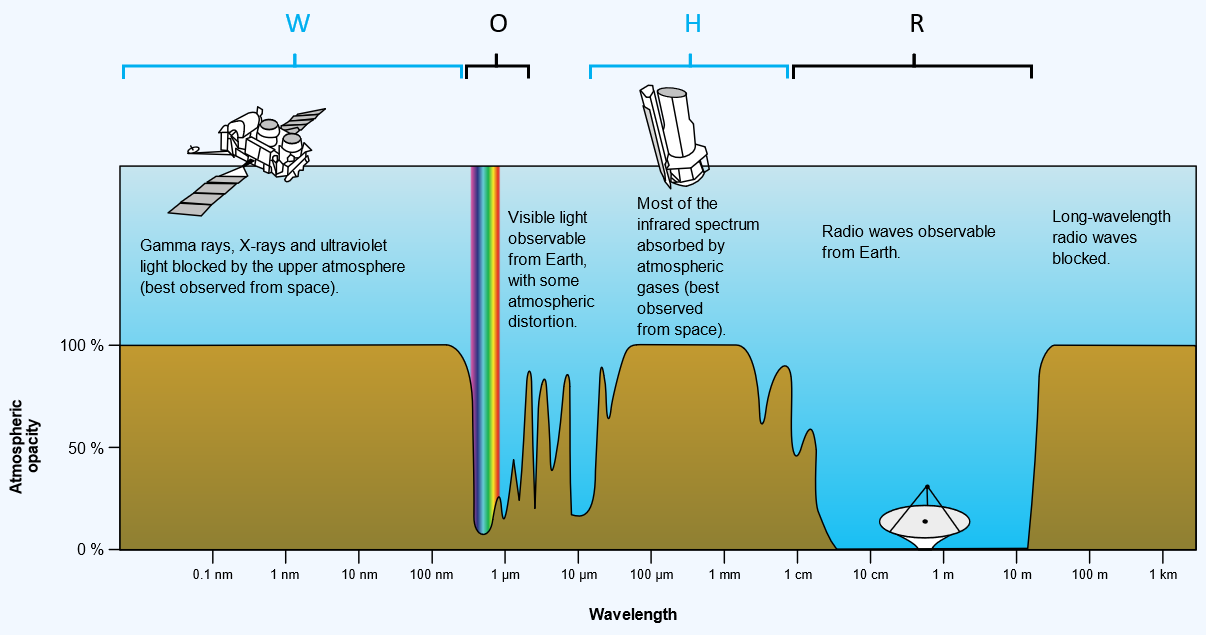
The areas marked W and H in Fig. 1 are therefore wavelength ranges in which observations can only be made from space or at very high altitudes (Sofia Stratospheric Observatory [1]). On the ground, the known ranges of visible light (Fig. 1 O) are suitable for classical astronomy with telescopes and the so-called radio window (Fig. 1 R), which extends from about 3 mm to 30 m wavelength.
Since electromagnetic waves travel at the speed of light, the wavelengths can be converted into frequencies by dividing the speed of light with the fixed value of 299,792,458 m/s by the wavelength. Frequencies can be converted into wavelengths accordingly.
The connection can be easily described via:
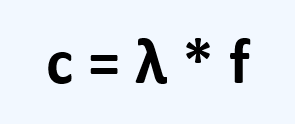
(with c= speed of light, λ=wavelength, f=frequency)
A wavelength of 30 cm (0.3 m) corresponds to a frequency of 999.308 MHz:
Calculation: 299.792.458 m/s / 0,3 m ≈ 999.308.193/s = 999.308.193 Hz = 999,308193 MHz
Radio telescopes therefore operate at wavelengths of 0.3 to 30 m and with frequencies between 10 MHz and 100 GHz.
In order to be able to receive the long wavelengths, correspondingly large apertures are needed – the telescopes must therefore have as large a collecting area as possible (=> dish diameter). For this reason, radio telescopes are usually larger receivers which, like the usual satellite dishes for television reception, have a large parabolically shaped primary mirror that concentrates the incident waves onto the focal point (primary focus). At this primary focus, the signal can be picked up directly, but it can also be reflected back into the secondary focus on the primary mirror via a second mirror (secondary mirror) attached there, where the corresponding receivers. This secondary focus is then much easier to reach for maintainance or change, especially with large systems.
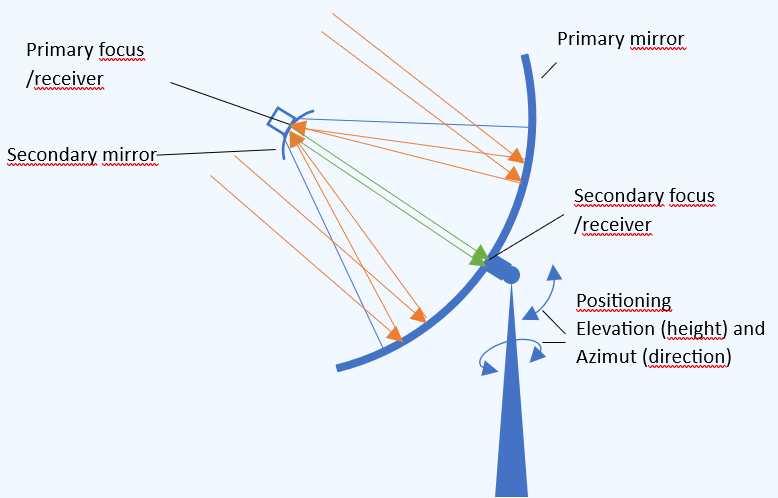
In order to obtain a higher resolution, several radio telescopes can be connected to observe the same astronomical object at the same time. The received signals from all participating radio telescopes are then superimposed on each other exactly in time with the help of atomic clocks, and the interferences enable a more accurate image of the observed object (interferometry and aperture synthesis [2]). Examples of such aperture syntheses or Interferometer can be found in systems like ALMA [3], SKA [4], VLBA [5], JVLA [6], WSRT [7] or the Korean KVN [8].
However, radio telescopes do not necessarily have to be movable, especially since the enormous mass (e.g. Effelsberg radio telescope 3200 t) of the large facilities stands in the way of movement. The currently largest radio telescope "FAST" in China [9] is an immovable receiving dish with a diameter of 500 m. The different positions of the objects to be observed are achieved by moving the receiver in the primary focus on a cable construction above the huge primary mirror, which is changed from spherical to parabolic shape in the area used. With this method, all objects above 50° elevation can still be reached – moving telescopes, on the other hand, can theoretically approach any point from horizon to 90° elevation.
What exactly do you observe with radio telescopes?
Radio radiation is long-wave electromagnetic radiation that we cannot perceive with the human eye. It can be divided into thermal and non-thermal radiation.
Thermal radiation occurs when elementary particles move quickly, i.e., when a body radiates heat, for example, because the particles are in rapid motion due to heat – the warmer, the faster. Max Planck described this heat radiation in his radiation law and showed that the hotter the radiation source (an idealized black radiator), the further the maximum of the radiation emission is in the short-wave range [10]. Wien's law of displacement can be derived from Planck's law of radiation – Wilhelm Wien had already derived the law before Planck and showed that the wavelength of the most intense radiation is inversely proportional to the temperature – if the temperature of a body doubles, the wavelength of the most intense radiation is halved [11].
Thermal radiation can be recognized by the fact that Planck's radiation law applies, i.e. there is a more or less linear dependence between radiation intensity and wavelength. This is very well recognizable, for example, in the appearance of very hot, blue-appearing and cooler, rather reddish-appearing stars. A very nice interactive presentation can be found on LEIFIphysik [12].
Interesting for radio astronomy is the "free-free-radiation", which is emitted by the free movement of particles in very hot gases. At correspondingly high temperatures between 5,000 and 20,000 K, gases are ionized (as plasma), i.e., electrons can move "freely" independently of the protons. However, if they then encounter another charged particle in their motion, they are attracted or repelled, which leads to a change in orbit – but the electron remains unbound, i.e. "free" again – hence the name "free-free radiation" or "bremsstrahlung". Every time a charged particle changes its orbit, radio radiation is released. In this way, hot gas clouds in the radio range can be detected very well via free-free radiation.
The cosmis background radiation at 2.725 K can be detected as thermal radio radiation. However, the maximum intensity is a wavelength of 1.06 mm and can therefore also only be observed at high altitudes or from space.
A special case for radio astronomy is thermal radiation from cosmic dust clouds, which radiate low heat (temperature between 10 and 100 K) and show a radiation maximum of about 290 μm. Since these wavelengths are in the submillimeter range, they can only be detected at high altitudes or in space due to atmospheric absorption – they can be used to detect the dust clouds around young stars.
In contrast to thermal radiation, non-thermal radiation is not linearly temperature-dependent. Here, in particular, the Synchrotron radiation is observed by radio astronomy. Synchrotron radiation is produced when fast, free electrons are deflected in their orbit by strong magnetic fields and directed onto spiral (so-called Spirelli) orbits – which is why it is also called "magnetobremsstrahlung". This happens mainly in the remnants of supernova explosions, which can thus be easily detected by radio astronomy. In addition, active galactic nuclei (AGNs) of galaxies and quasars are also powerful synchrotron emitters, so that they can be observed or detected very well with radio astronomical means. The emergence of tw. very strong cosmic magnetic fields is not yet fully understood.
A radio emission that is also non-thermal is the spectral emission of gas. In this process, gases emit radio radiation after excitation, e.g. by photons, because they experience transitions of discrete excitation states at different levels. Many astronomers are familiar with the observation of the Hα line of hydrogen, for example, which is caused by the electron being moved to a higher energy level (in this case, a change in the principal quantum number n) and emits light at a wavelength of 656.281 nm when falling back. In radio astronomy, the transition of the so-called hyperfine structure is observed. [13] , in which the stimulation of the spin quantum number (s) of hydrogen is changed and the electron changes from the antiparallel to the parallel spin with respect to the proton by a spin flip. This state is slightly more energetic than the ground state (difference 5.88 μeV) and very stable – the half-life is about 11 million years. However, if the electron falls back into the ground state (antiparallel spin), the energy is radiated in the form of radio radiation at a frequency of 1420.405 MHz. Via the relationship between frequency and wavelength described above, this frequency corresponds to a wavelength of about 21 cm – the 21 centimeter line of hydrogen..
With the help of the 21 cm line, the distribution of hydrogen in space can be observed by radio astronomy. In addition, the displacement of the discrete 21 cm line by Doppler effects can also show movements of gas clouds relative to the observation site, so that e.g. the rotation of a gas cloud becomes visible.
Other gases also emit specific line radiation – mainly due to the rotation of the atoms or molecules. An overview of the corresponding. Frequencies or wavelengths of gas atoms and molecules observable by radio astronomy can be found, for example, in chap. 5.8 in the CRAF Handbook for Radio Astronomy of the CRAF (COMMITTEE ON RADIO ASTRONOMY FREQUENCIES) [14], which also provides valuable information on radio astronomy.
Sources:
[1] Stratospheric Observatory for Infrared Astronomy https://en.wikipedia.org/wiki/Stratospheric_Observatory_for_Infrared_Astronomy
[2] Aperture synthesis https://www.spektrum.de/lexikon/physik/apertursynthese/660
[3] ALMA https://www.eso.org/public/germany/teles-instr/alma/
[4] SKA https://www.mpifr-bonn.mpg.de/forschung/fundamental/ska
[5] VLBA https://public.nrao.edu/telescopes/vlba/
[6] JVLA https://de.wikipedia.org/wiki/Very_Large_Array
[7] WSRT https://de.wikipedia.org/wiki/Westerbork_Synthese_Radio_Telescoop
[8] KVN https://radio.kasi.re.kr/kvn/maJn.php
[9] FAST https://fast.bao.ac.cn/
[10] Planck's law https://en.wikipedia.org/wiki/Plancks_law
[11] Wien's displacement law https://en.wikipedia.org/wiki/Wiens_displacement_law
[12] Black-body radiation https://www.leifiphysik.de/optik/elektromagnetisches-spektrum/ausblick/temperaturstrahlung-und-strahlungsgesetze
[13] Hyper fine structure of Hydrogen https://en.wikipedia.org/wiki/Hydrogen_line
[14] CRAF Handbook for Radio Astronomy https://craf.eu/wp-content/uploads/2015/02/CRAFhandbook3.pdf